Gas-phase Structures of Biomolecular Ions
Native electrospray ionization produces intact biomolecules and their complexes in the gas phase, but it is often unclear whether these maintain their solution-phase native conformation. We are investigating this fundamental question:
Fluorescence spectroscopy
We are using fluorescence spectroscopy as an orthogonal method to MS to study mass selected, trapped ions. The emission spectrum of a fluorophore can be utilized directly to probe its chemical and physical environment, for instance the pH of a droplet or the encapsulation in a macromolecule. In combination with a secondary fluorophore, Förster Resonance Energy Transfer (FRET), i.e., radiation-free transfer of energy from an absorbing donor to an emitting acceptor fluorophore, can be empoyed to deduce inter-dye distances and therefore to obtain information on the structure. The coupling of fluorescence spectroscopy and trapped ion mass spectrometry is challenging due to the low gas-phase ion density.
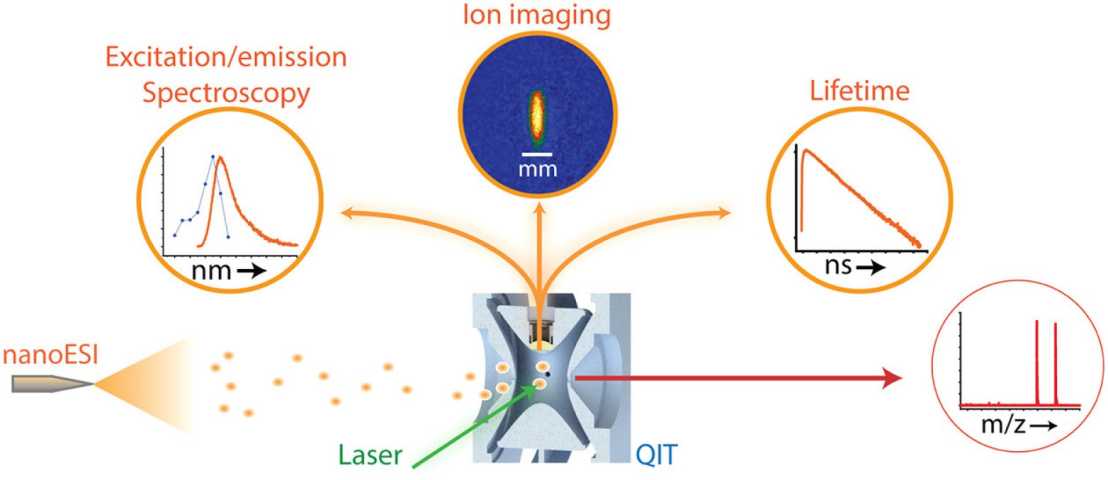
Quadrupole ion trap
A setup to for laser-induced fluorescence experiments on trapped, mass-selected biomolecular ions has been developed. It consists of a quadrupole ion trap (QIT) mass spectrometer modified in-house to enable optical access to trapped ions for excitation with laser pulses and collection of the emitted fluorescence. An interface to differential ion mobility spectrometry (DMS) also allows selection of certain conformations before entering the QIT. A unique aspect of this apparatus is that it allows both wavelength- and time-resolved fluorescence measurements. The light source in the experiments is a pulsed (~100 fs pulses), tunable (690-1040 nm) titanium:sapphire laser (Mai Tai HP, Spectra-Physics, Santa Clara, USA), which is frequency doubled to access the UV-Vis wavelength range (345-520 nm). The tunability of laser light in this wavelength enables us to probe the spectroscopic properties of biologically relevant chromophores.
Current Scientific projects:
The way in which an analyte is ionized by ESI can depend on the distribution of ionizable groups within the analyte, its size and shape, the composition of the solvent used for ESI, and on whether other additives (e.g., so-called supercharging agents) are present. We have developed fluorescence-based methods to study the mechanisms of the ESI process, using solvatochromic fluorescent probes. Dispersed and time-resolved fluorescence measurements of the fluorescing species present inside the electrospray plume can provide snapshots of their chemical environments, including whether they are solvated or desolvated, their ionization state, and whether they have retained their solution-phase structures. These experiments promise to give not only fundamental insights into the ESI process but will render the ESI method a more versatile tool in analysis.
An important question in the biological mass spectrometry community is whether, and under what conditions, the ionized biological molecules inside the mass spectrometer resemble their states present in solution. While in the condensed phase one can obtain detailed NMR and X-ray crystallographic structural data, not a single technique exists that yields such high-resolution structural information in the gas phase. Our group is developing fluorescence-based structural probes to examine the structures of trapped gaseous biomolecular ions and their noncovalent complexes. Among the techniques available, FRET is the most intriguing, as it provides a way to extract nanometer-scale distance information in gaseous biomolecules. For these experiments, we use a quadrupole ion trap and a FT-ICR mass spectrometer, which have been modified in-house for fluorescence measurements. Ion mobility is also employed for conformational selection of analytes.
We recently reported the first evidence of transition metal ion FRET (tmFRET) in the gas phase and its application to measure short (10–40 Å) biomolecular backbone distances. The measured FRET efficiencies in rhodamine dye (donor) labeled helical peptides complexed with Cu2+ ions (acceptor) decreased with increasing donor - acceptor distances, confirming the occurrence of tmFRET. The distances estimated for similar peptide sequences from the FRET efficiencies were consistently longer in the gas phase compared to those reported in solution, indicating an expanded structure and a possible loss of helicity. Additionally, experiments are performed inside an ESI plume to probe the transition from solution phase to gas phase and its effects on the biomolecular structure.
Other gas-phase fluorescence spectroscopic techniques:
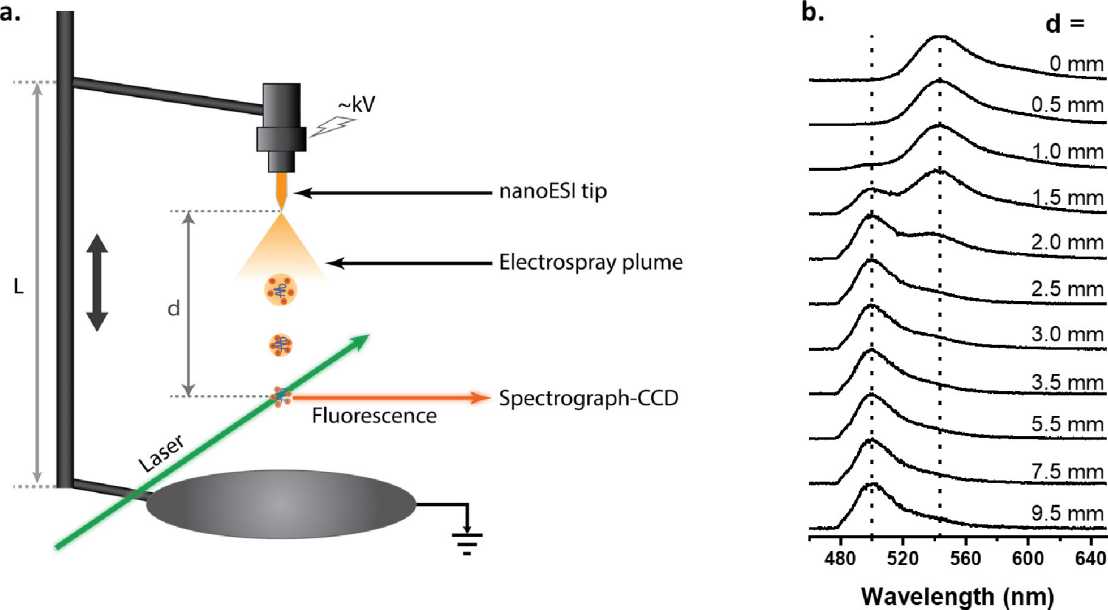
Electrospray produces small charged droplets; these droplets undergo repeated cycles of fission and evaporation, eventually resulting in the release of charged analyte. The mechanisms that govern ion production remain difficult to study. Mechanistic insights promise not only to further our fundamental understanding of the ESI process, but will facilitate optimization of ion formation as well. Laser-induced fluorescence experiments directly in the electrospray plume, provide snapshots of the conformations, electronic structures, and dynamics of electrosprayed analytes both within charged droplets, and after desolvation.
Staff: Dr. Ri Wu, Lukas Benzenberg, Dr. Kim Greis