Nanoscale Analysis
external pageTip-enhanced Raman Spectroscopy (TERS)call_made is a powerful analytical technique for surface molecular imaging at the nanoscale under ambient conditions. It combines high spatial resolution of scanning probe microscopy (SPM) with the high chemical sensitivity and specificity of surface-enhanced Raman spectroscopy (SERS). Our team focuses on the further development of this technique and its application in the material, chemical and biological sciences, with the aim to turn it into a versatile analytical tool for nanoscience and nanotechnology. [NOTE: this is NOT nanomaterial/nanoparticle based analytical chemistry]
Raman spectroscopy is a well-established analytical tool that provides fingerprint vibrational information from the molecules under investigation. However, Raman scattering is a relatively weak effect compared to, for e.g., fluorescence. SERS overcomes this issue using localized surface plasmon resonance and offers several orders of magnitude improvement in sensitivity via plasmonic signal enhancement. However, the (often random) heterogeneity of SERS substrates leads to a significant variation in plasmonic signal enhancement across the sample surface limiting its range of applications especially nanoscale molecular imaging. In TERS, the signal enhancing structure is reduced to a single 'SERS hotspot' created at the end of a sharp metallic tip, which can be accurately positioned in contact with a surface using either an atomic force microscope (AFM) or a scanning tunnelling microscope (STM). This approach, external pagepioneered in our laboratory in 2000call_made, provides the possibility to perform hyperspectral molecular imaging with greater than 10 nm spatial resolution by scanning the local 'SERS hot spot' over the sample surface.
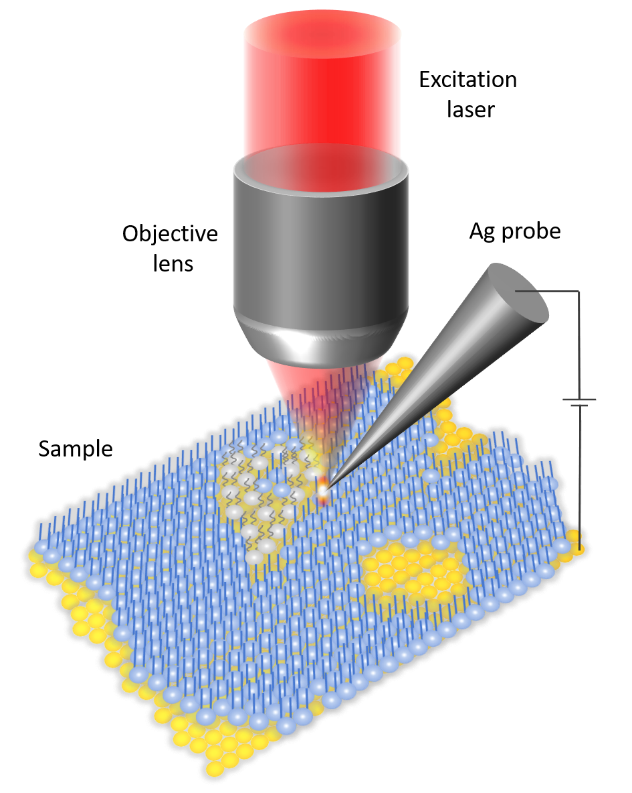
A typical TERS instrument combines a confocal laser scanning Raman microscope with an AFM or STM and contains a set-up for precise alignment of a customized metallic SPM probe with the excitation laser spot. A piezo-controlled movable sample stage is used to perform hyperspectral Raman imaging of the sample surface in nm size steps. TERS systems can be based on an inverted (bottom illumination) or upright (top illumination) optical microscope (both available in our laboratory) and in most cases the same objective is used for excitation and collection of Raman signals. Figure 1 schematically shows the basic working principle of a TERS setup combining a Raman microscope with a STM in upright geometry.
The general aim of our research is to turn TERS into a versatile analytical technique for surface science, with a particular emphasis on reproducible molecular imaging at the nanoscale under ambient conditions.
Current Scientific Projects:
Using reproducible TERS imaging we study a broad range of materials, including self-assembled monolayers, 2D polymers, single-layer graphene, polymer-blend thin films, photovoltaic materials etc. Our aim is to develop TERS into a reliable nanoanalytical tool for routine analysis of molecular thin-film and nanostructured materials.
We investigate nanoscale defects and heterogeneities in soft 2D materials such lipid membranes. Biological membranes and their components (lipids, proteins, glycans) are notoriously difficult to study using TERS due to their rather low Raman cross-section. Furthermore, extended laser irradiation and intense local fields often lead to material decomposition. Our goal is to study the degradation pathways and find methodological innovations to overcome these challenges. Performing TERS experiments in liquids (the natural environment for membranes) and using protected tips are some of the novel approaches being developed.
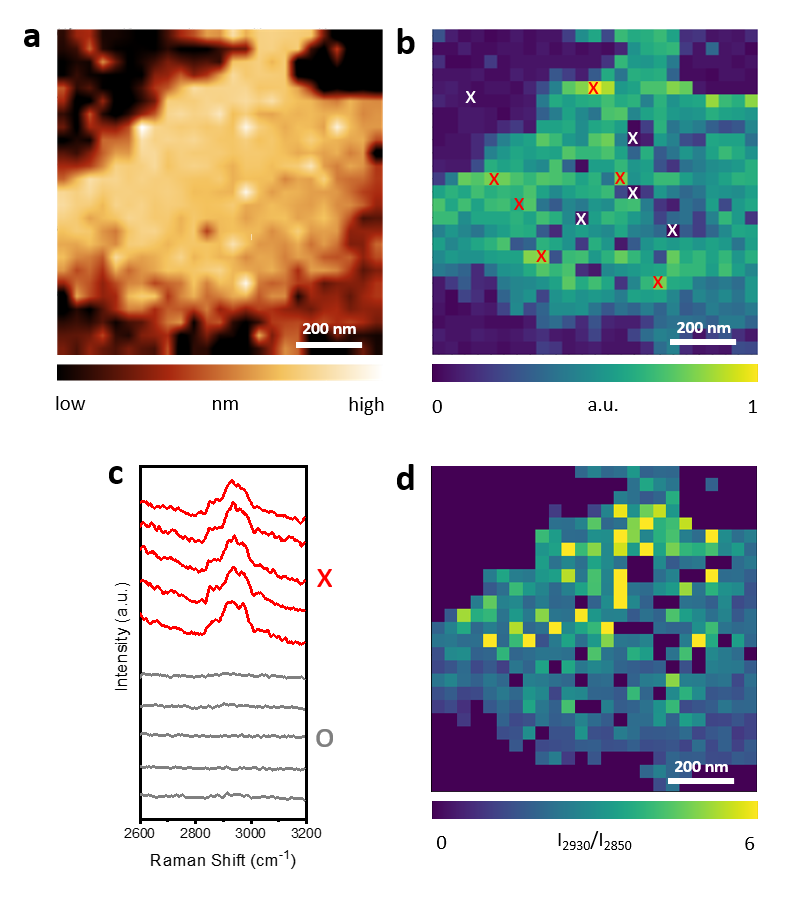
Figure 2. external pageNanoscale Chemical Imaging of a Supported Lipid Monolayer using TERS.call_made (a) STM topography image of a DPPC monolayer sample on Au(111) surface prepared via Langmuir Blodgett transfer. (b) TERS image of the C-H stretching (2800–3000 cm-1) intensity measured in the area shown in (a). TERS image reveals a significant molecular heterogeneity in the DPPC monolayer. (c) TERS spectra measured at the locations of high signal intensity marked as “red x” and locations of low signal intensity marked as “white x” in (b). No TERS signal is observed at the locations of white x indicating the presence of holes within the lipid monolayer. (d) TERS image of the ratio of 2850 cm-1 and 2930 cm-1 band intensity in the C-H stretching region. Higher ratio represents a higher level of disorder in the DPPC monolayer.
Visualization of chemical reaction at catalytically active sites is the key to rational design of novel catalyst materials. With single molecule sensitivity and nanoscale spatial resolution, TERS is ideally suited to meet this challenge. In our research, we apply TERS to study both model and real-life catalytic systems including plasmon-driven photocatalytic reactions, bimetallic catalysts, and ZSM-5 crystals.
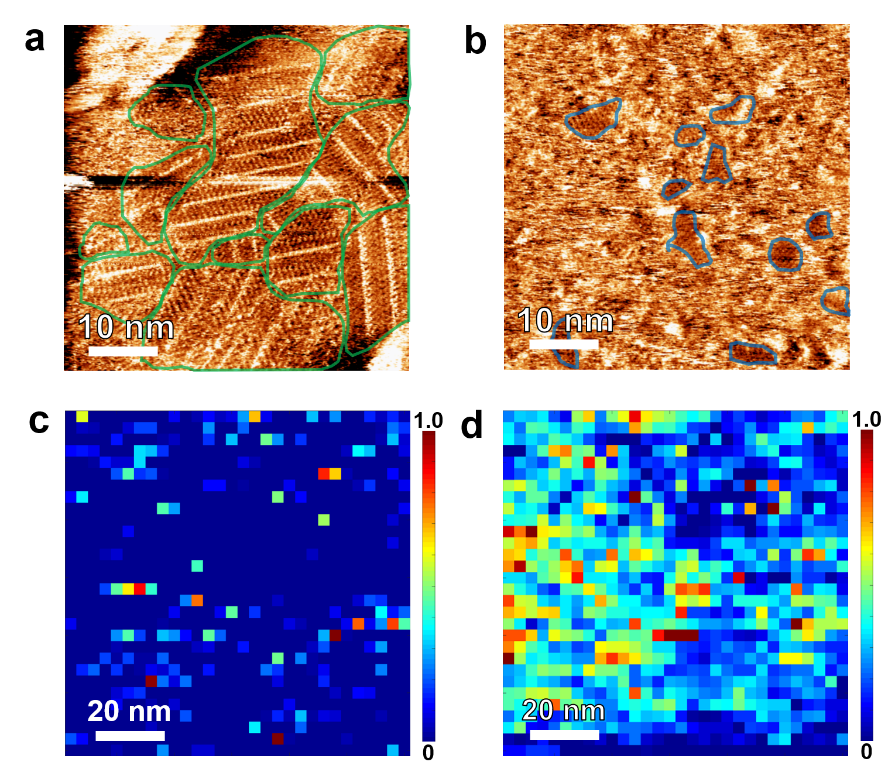
Figure 3. external pageMolecular-Level Insights on Reactive Arrangement in On-Surface Photocatalytic Coupling Reactions Using TERS.call_made STM images of 4-NTP adlayer at air/Au interface prepared by (a) immersing Au substrate in a 4-NTP solution for 24 h (immersion sample) and (b) drop-casting 4-NTP solution on Au surface for 60 s (drop-cast sample). A close-packed ordered molecular arrangement is observed on the immersion sample whilst the drop-cast sample mostly contains a disordered arrangement of 4-NTP molecules. High-resolution TERS images of the photocatalytic conversion of 4-NTP → DMAB measured on (c) immersion and (c) drop-cast samples. Correlation of molecular-resolution STM and high-resolution TERS imaging indicate that a close-packed molecular arrangement is unfavorable for efficient conversion of 4-NTP→DMAB. DFT modeling revealed a combination of steric hindrance effect and energetic barrier as the predominant cause of lower reaction efficiency in the ordered phase of the 4-NTP adlayer.
A major focus of our research is understanding and continuous improvement of TERS measurements, instrumentation, and methodology. For this, we perform comparison studies of TERS measurements from different TERS geometries, probes, and SPM modes. Furthermore, since metallic tips are the key to a successful TERS experiment, improving the sensitivity, reproducibility, robustness, and durability of TERS probes is also an important part of our research.